It’s not terribly hard to turn me into a squealing fangirl. One of the ways is to agree with me eloquently and/or share my pet peeves. Another is to give me lightbulb moments. A third is to disagree with me in a well-reasoned, intelligent way. And finally, if I see you thoughtfully examining your own thinking, you are awesome by definition. Michaël Manuel’s monster review of body symmetry and polarity in animals (Manuel, 2009) did all of the above.
(In case you wondered, that means a long, squeeful meandering >.>)
Manuel writes about the evolution of two fundamental properties of animal body plans [1]: symmetry and polarity. You probably have a good intuitive understanding of symmetry, but here’s a definition anyway. An object is symmetrical if you can perform some transformation (rotation, reflection, shifting etc.) on it and get the same shape. Polarity is a different but equally simple concept – it basically means that one end of an object is different from the other, like the head and tail of a cat or the inner and outer arcs of a rainbow.
I can’t say that I’d thought an awful lot about either before I came across this review, so it’s not really surprising that I had lightbulbs going off in my head left and right while I was reading it. Because I didn’t think deeply about symmetry and polarity and complexity, I basically held the mainstream view I – and, I suspect, most of the mainstream – mostly picked up by osmosis.
That meant I fell victim to my own biggest pet peeve big time – I believed, without good reason and without even realising, that the body plan symmetries of major lineages of living animals represented successive increases in complexity. Sponges are kind of asymmetrical, cnidarians and ctenophores are radially symmetrical, and bilaterians such as ourselves have (more or less) mirror image symmetry, and these kinds of symmetry increase in complexity in this order. Only… they aren’t, and they don’t.
It turns out that this guy not only shares my pet peeve but uses it to demolish my long-held hidden assumptions. Double fangirl points!
Let there be light(bulbs)!
Problem number one with the traditional view – aside from ignoring that evolution ain’t a ladder – is that the distribution of symmetry types among animals is a little more complicated. Most importantly, most kinds of sponges are not asymmetrical. Most species may be, but that’s not the same thing. You see, most sponge species are demosponges, which make up only one of the four great divisions among sponges. Demosponges do have a tendency towards looking a bit amorphous, but the other three – calcareous sponges, glass sponges and homoscleromorphs – usually are some kind of symmetrical. All in all, the evidence points away from an asymmetrical animal ancestor. (Below: calcareous sponges being blatantly symmetrical, from Haeckel’s Kunstformen der Natur.)

The second problem is that my old view ignores at least one important kind of symmetry. Some “radially” symmetrical animals are actually closer to cylindrical symmetry. To understand the difference, imagine rotating a brick and a straight piece of pipe around their respective long axes. You can rotate the pipe as much or as little as you like, it’ll look exactly the same. In contrast, the only rotation that brings the brick back onto itself is turning it by 180° or multiples thereof. A pipe, with its infinitely many rotational symmetries, is cylindrically symmetrical, while the brick has a finite number of rotational symmetries [2], making it radially symmetrical.
Problem number three is that bilateral symmetry is actually no more complex than radial symmetry! What does “complexity” mean in this context? Manuel defines it as the number of coordinates required to specify any point in the animal’s body. In an animal with cylindrical symmetry, you only need a maximum of two: where along the main body axis and how far from the main body axis you are. Everything else is irrelevant, since these are the only axes along which the animal may be polarised. (Add any other polarity axis, and you’ve lost the cylindrical symmetry.)
Take a radially symmetrical creature, like a jellyfish. These also have a main rotational axis and an inside-outside axis of polarity. However, now the animal’s circumference is also divided up into regions, like slices in a cake. How does a skin cell around a baby jelly’s mouth know whether it’s to grow out into a tentacle or contribute to the space between tentacles? That is an extra instruction, an extra layer of complexity. We’re up to three. (Incidentally, here’s some jellyfish symmetry from Haeckel’s Kunstformen. [Here‘s photos of the real animal] A big cheat he may have been, but ol’ Ernst Haeckel certainly had an eye for beauty!)

And with that, jellies and their kin essentially catch up to the basic bilaterian plan. Because what do you need to specify a worm? You need a head-to-tail coordinate, you need a top-to-bottom one, and you need to say how far from the plane of symmetry you are. Still only three! Many bilaterians, including us, added a fourth coordinate by having different left and right sides, but that’s almost certainly not how we started when we split from the cnidarian lineage. (Below: radial symmetry doesn’t hold a monopoly on beauty! Three-striped flatworm [Pseudoceros tristriatus] by wildsingapore.)

Not only that, but Manuel argues that there’s very little evidence bilateral symmetry evolved from radial symmetry. By his reckoning, the most likely symmetry of the cnidarian-bilaterian common ancestor was cylindrical and not radial (more on this later, though). Thus the (mostly) radial cnidarians and the (mostly) bilateral bilaterians represent separate elaborations of a cylinder rather than stages in the same process.
There were a bunch more smaller lightbulb moments, but I’m already running long, so let’s get on to other things.
Respectful disagreement
I think my disagreements with Manuel’s review are more of degree than of kind. Our fundamental difference of opinion comes back to the symmetries of various ancestors and the evidence for them. He argues that key ancestors in animal phylogeny – that of cnidarians + bilaterians, that of cnidarians + bilaterians + ctenophores, and that of all animals – were cylindrical. (Below is the reference tree Manuel uses for his discussion, with symmetry types indicated by the little icons.)
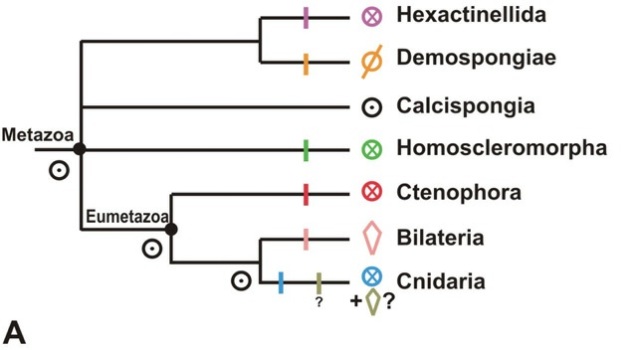
I think he may well be correct in his conclusions, but I’m not entirely comfortable with his reasons. For example, he infers that the last common ancestor of cnidarians and ctenophores was cylindrical. One of his main arguments is that the repeated structures that “break up the cylinder” to confer radial symmetry are not the same in these two phyla. I think this is an intelligent point a smart guy who knows his zoology would make, so disagreement with it becomes debate as opposed to steamrolling [3].
Why I still disagree? As I said, it comes down to degrees and not kinds. Manuel considers the above evidence against a radially symmetrical common ancestor. I consider it lack of evidence for same. The situation reminds me of Erwin and Davidson (2002), which is also one of my favourite papers ever. They raise perhaps the most important point one could make about comparative developmental genetics: homologous pathways could have been present in common ancestors without the complex structures now generated by those pathways being there. Likewise, I think, radial symmetry could have been there in the common ancestor of cnidarians and ctenophores while none of the complex radially symmetrical structures (tentacles, stomach pouches, comb rows etc.) in the living animals were. Perhaps there were simpler divisions of cell types or whatnot that gave rise to the more overt radial symmetry of jellyfish, sea anemones and comb jellies.
In a related argument, Manuel discusses the homology (or lack thereof) of the dorsoventral axis in bilaterians and the so-called directive axis in sea anemones. Sea anemones actually show hints of bilateral symmetry, which prompted some authors (e.g. Baguñà et al., 2008) to argue that this bilateral symmetry and ours was inherited from a common ancestor (i.e. the cnidarian-bilaterian ancestor was bilateral).
I agree with Manuel that the developmental genetic evidence for this is equivocal at best. I even agree with him that developmental genetics isn’t decisive evidence for homology even if it matches better than it actually does in this case. But again, once the genetic evidence is dismissed as inconclusive, he relies on the non-homology of bilaterally symmetrical structures to conclude non-homology of bilateral symmetry. Again, I think this is a plausible but premature inference. Since I’m not sure whether homology or independent origin of bilateral symmetry is the better default hypothesis in this case, and I don’t think the evidence for/against either is convincing, I actually wouldn’t come down on either side as of yet.
But I can see his point, and that’s really cool.
Why else you’re awesome, Michaël Manuel…
Because you have a whole rant about “basal lineages”. I grinned like a maniac throughout your penultimate paragraph. Incidentally, you might have given me another favourite paper – anything with “basal baloney” in its title sounds like it’s worth a few squees of its own!
Because you apply critical thinking to your own thinking. See where we disagreed, non-homology of structures vs. symmetries, evidence against vs no evidence for, and all that? After you made the argument from non-homology of structures, I expected you to leave it at that. And you didn’t. You went and acknowledged its limitations, even though you stood by your original conclusions in the end.
Because you reminded me that radial symmetry is similar to metamerism/segmentation. I’d thought of that before, but it sort of went on holiday for a long time. Connections, yay!
Because you were suspicious about sponges’ lack of Hox/ParaHox genes. And how right you were!
*
Phew, that turned out rather longer and less coherent than I intended. And I didn’t even cover half of the stuff in my notes. I obviously really, really loved this paper…
***
[1] Or any body plan, really…
[2] Astute readers might have noticed that a brick has more than one axis of symmetry, plus several planes of symmetry as well. So it’s not only radially but also bilaterally symmetrical. The one thing it certainly isn’t is cylindrical 😉
[3] Not to say I don’t enjoy steamrolling obvious nonsense, but I also like growing intellectually, and steamrolling obvious nonsense rarely stretches the mind muscles…
***
References:
Baguñà J et al. (2008) Back in time: a new systematic proposal for the Bilateria. Philosophical Transactions of the Royal Society B 363:1481-1491
Erwin DH & Davidson EH (2002) The last common bilaterian ancestor. Development 129:3021-3032
Manuel M (2009) Early evolution of symmetry and polarity in metazoan body plans. Comptes Rendus Biologies 332:184-209